All GRE Subject Test: Biochemistry, Cell, and Molecular Biology Resources
Example Questions
Example Question #361 : Gre Subject Test: Biochemistry, Cell, And Molecular Biology
In a species of ant, black coloration is dominant to white. A scientist is operating under the assumption that this gene follows basic Mendelian principles; however, after crossing two heterozygotes he obtained a ratio of 2:1 of dominant to recessive offspring. Which of the following could explain this result?
The alleles display incomplete dominance
The homozygous recessive phenotype is lethal
The homozygous dominant phenotype is lethal
The alleles display codominance
The homozygous dominant phenotype is lethal
The only answer that properly explains a 2:1 ratio is if the homozygous dominant phenotype is lethal. In our punnett square, this would give a two-thirds chance for a heterozygous offspring and a one-third chance for a recessive offspring. We know the recessive phenotype is not lethal because homozygous recessive offspring were produced.
Parents: Aa x Aa
Offspring genotypes: AA, Aa, Aa, aa
Offspring phenotypes: lethal, dominant, dominant, recessive (only three live offspring produced)
Offspring ratio: 2 dominant to 1 recessive
There is also no evidence that codominance or incomplete dominance is present. If black and white spotted offspring or gray offspring were produced then these theories would have merit.
Example Question #15 : Molecular Biology And Genetics
A scientist has been working with a new species of plant. He has found that there are two separate genes, which segregate according to standard Mendelian genetics, that are capable of producing the same phenotype. A single dominant allele from either gene confers red coloration of the plant's flowers. Without any dominant alleles the flowers are white. If he crosses two plants heterozygous for both traits, what will be the resulting phenotypic ratios of the offspring?
This problem requires a standard dihybrid cross. The crossed genotypes are AaBb x AaBb. This results in a phenotypic ratio of 9 dominant for both traits, 3 dominant for a single trait, 3 dominant for the other trait, and 1 recessive for both traits. In this cross, it will result in: 9 AxBx, 3 Axbb, 3 aaBx, and 1 aabb.
Since we know that the genes are both capable of making the red coloration we actually need to add together all of the choices that contain at least a single dominant allele. Essentially, AxBx, Axbb, and aaBx all show the exact same phenotype. This leaves us with a 15:1 ratio of red to white flowers.
Example Question #2 : Help With Mendelian Inheritance
A child is curious to know what his blood type is, but he only knows his parents' blood types. If his mother had blood type A and his father had blood type AB, what are the potential blood types the child might have?
A or B
A, B, or O
A or AB
A, B, or AB
A, B, or AB
We are not given the mother's full genotype in the question; she could reasonably carry two A alleles, or an A allele and a recessive O allele. We know that the father must carry one copy of the A allele and one copy of the B allele.
Two punnett squares can answer this question, corresponding to the two possible maternal genotypes: one crossing AA x AB and the other crossing AO x AB. From the first cross there is a 50% chance of blood type A versus 50% chance of blood type AB (half AA and half AB). The second cross shows that there is a potential chance of 50% for type A, 25% for type AB, and 25% for type B (one AA, one OA, one AB, and one OB).
Based on these possibilities, the child could have blood type A, B, or AB. The child cannot have blood type O.
Example Question #2 : Help With Mendelian Inheritance
A breeder performs a standard dihybrid cross between two plants that are heterozygous for both traits in question. How many unique genotypes could be present in the resulting offspring?
Sixteen
Four
Fifteen
Nine
Nine
There are nine distinct genotypes present after a standard dihybrid cross. This question can easily be answered by setting up a Punnett square (AaBb x AaBb) and counting the number of unique genotypes present after doing the cross. The numbers also conveniently work out that however many offspring display the dominant phenotype is equal to the number to of genotypes present (this is true for monohybrid and trihybrid crosses as well).
Example Question #4 : Help With Mendelian Inheritance
A scientist is performing a monohybrid homozygous cross: tall plants crossed with short plants. What fraction of the F2 generation are homozygous tall?
A monohybrid cross between two homozygous plants would involve a parental generation that looked like this: SS (tall) x ss (short). The F1 generation would produce only heterozygous tall plants (Ss). The F2 generation would produce offspring from the following cross: Ss x Ss. A punnett square would reveal that the F2 generation would have 25% homozygous tall (SS), 50% heterozygous tall (Ss), and 25% homozygous short plants. Note that we do not need information regarding which trait is dominant in this case, and we would still get the correct answer if we took short as the dominant phenotype.
Example Question #3 : Inheritance
In apple trees, the allele for white blossoms is dominant over the allele for pink blossoms. Two trees heterozygous for this gene are crossed. What is the phenotype ratio of the offspring?
3 white : 1 pink blossom
All white blossoms
1 white : 3 pink blossom
1 white : 1 pink blossom
All pink blossoms
3 white : 1 pink blossom
The crossing of two heterozygous parents will yield 1 homozyougs dominant offspring, 1 homozygous recessive offspring and 2 heterozygous offspring. Since white blossoms is the dominant allele, the heterozygous offspring will be white leading to a phenotypic ratio of 3 white : 1 pink blossom.
Example Question #21 : Molecular Biology And Genetics
Assume complete dominance inheritance for the following question.
A pure-breeding red flower is mated with a pure-breeding white flower. All offspring are red in color; this is the F1 generation. Two of these offspring flowers are then mated with one another, and have F2 offspring.
Which of the following is true of the F2 offspring?
All flowers will be white.
All flowers will be red.
There will be more red flowers than white flowers.
There will be a 50/50 ratio of red to white flowers.
There will be more red flowers than white flowers.
Since we had pure-breeding parents (also known as homozygotes for their respective colors), we can safely say the F1 offspring are heterozygotes and have a red allele and a white allele. When crossing these offspring with one another, we will expect to get a 3:1 ratio of red to white flowers. Not all flowers will be red, but 75% of the flowers will be.
Shown below is the punnett square that reflects this conclusion. (Note that "A" represents a red allele and "a" represents a white allele):
A a
A AA (red) Aa (red)
a Aa (red) aa (white)
Example Question #1 : Help With Hardy Weinberg
A population of insects exists in which black coloration is dominant to white. If there are 64 black insects and 36 white insects in the population, what is the recessive allele frequency?
We can use the white insect population to figure out our recessive allele frequency if we use the Hardy-Weinberg equations:
From this equation we know that is actually the frequency of our homozygous recessive genotype. We can determine the value of this genotypic frequency based on the information in the question. Any white insects must be homozygous recessive.
Solve for the recessive allele frequency.
Example Question #2 : Help With Hardy Weinberg
A population of lizards is shown to have 36 members that are homozygous dominant, 48 members that are heterozygous, and 16 members that are homozygous recessive for a particular trait. The population displays Hardy-Weinberg equilibrium. What are the allele frequencies present in this population?
To solve this question, we must use the Hardy-Weinberg equations:
There are two ways to solve this problem. The easier way is to use the second Hardy-Weinberg equation. We are told that the population is in Hardy-Weinberg equilibrium, so the observed genotype frequencies equal the expected genotype frequencies. Each term in the second Hardy-Weinberg equation can be used in coordination with the given phenotypic frequencies.
The dominant allele frequency is 0.6 and the recessive allele frequency is 0.4.
The second method involves using our population and the total number of alleles. Since our population totals to 100, we have a total of 200 alleles (two alleles per member in the population. Next, to get the frequencies we simply have to divide the total number of a single allele by the total number of alleles in the population. For the dominant allele frequency it would look like this:
We can then get the recessive allele frequency from the first Hardy-Weinberg equation:
Both methods result in the same answer.
Example Question #21 : Molecular Biology And Genetics
A population of beetles exists in which black coloration is dominant to white. The allele frequencies of the population were originally o.4 for the dominant allele and 0.6 for the recessive allele. A predator was introduced that selectively ate the white beetles. The new population consists of 36 homozygous dominant black beetles, 48 heterozygous beetles, and 16 white beetles. What are the new allele frequencies?
The original allele frequencies are actually superfluous information that we will not need to use in our calculation. We are given the populations of each genotype, so we can use the Hardy-Weinberg equations to solve for the allele frequencies.
In the second equation, gives the frequency of the homozygous dominant phenotype and
gives the frequency of the homozygous recessive phenotype. Using the population ratios from the question we can solve for these values to find the allele frequencies.
The problem could also be solved by summing the total number of alleles, and dividing the total of each individual allele by this number. Keep in mind that each individual carries two alleles.
Certified Tutor
Certified Tutor
All GRE Subject Test: Biochemistry, Cell, and Molecular Biology Resources
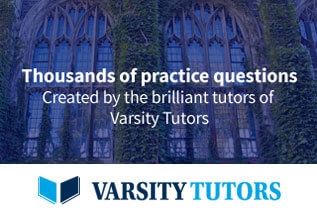