All Biochemistry Resources
Example Questions
Example Question #57 : Citric Acid Cycle
How many molecules of and
, respectively, are produced during the conversion of citrate to oxaloacetate?
. . .
. . .
. . .
. . .
. . .
During the step in which one of the carbons of isocitrate is lost as carbon dioxide, one molecule is also produced. This reaction is catalyzed by isocitrate dehydrogenase. This leaves a five-carbon molecule known as alpha-ketoglutarate. In the next step, alpha ketoglutarate dehydrogenase acts upon alpha-ketoglutarate and a carbon is lost as carbon dioxide and another molecule of
is produced. Later in the cycle, succinate dehydrogenase catalyzes the conversion of succinate to fumarate. This reaction produces one molecule of
. The enzyme fumarase then converts fumarate to malate. The final step in the citric acid cycle is the regeneration of oxaloacetate from malate. Malate dehydrogenase catalyzes this reaction, which produces the third molecule of
. Note that this is for one turn of the citric acid cycle i.e., for one molecule of acetyl-CoA. Each molecule of glucose yields two molecules of acetyl-CoA via glycolysis and pyruvate dehydrogenase complex.
Example Question #4 : Other Citric Acid Cycle Concepts
Which of the following is true about the citric acid cycle?
In eukaryotes, the cleavage of citrate to form acetyl-CoA takes place in the cytosol
All organisms except archaea have a complete citric acid cycle
Although some citric acid cycle intermediates are precursors to other biosynthetic pathways, no other biosynthetic pathways produce citric acid cycle intermediates
Anaplerotic reactions remove metabolites from the citric acid cycle
In eukaryotes, the cleavage of citrate to form acetyl-CoA takes place in the cytosol
Some catabolic pathways do indeed make citric acid cycle intermediates; for example, plants and bacteria use phospoenolpyruvate carboxylase to create oxaloacetate from phosphoenolypyruvate. Anaplerotic reactions refill the citric acid cycle with intermediates, rather than remove them. Some archaea have a complete citric acid cycle; it is the bacteria that mostly do not have a complete cycle. In eukaryotes, citrate cleavage does indeed take place in the cytosol; that citrate is transported to the cytosol from mitochondria, and the acetyl-CoA can be used for fatty acid synthesis.
Example Question #58 : Citric Acid Cycle
Which of the following most clearly states the main purpose of the citric acid cycle?
Breaks down fatty acids through a cyclical series of steps that produces acetyl-CoA
Helps produce energy for eukaryotic cells when oxygen is abundant
Contains hydrolytic enzymes to degrade and recycle organic compounds
Helps produce energy for prokaryotic or eukaryotic cells via anaerobic fermentation
Takes in ammonia, a byproduct of amino acid catabolism, and converts it into harmless urea
Helps produce energy for eukaryotic cells when oxygen is abundant
The citric acid cycle is a series of reactions that occur within the matrix of the mitochondria. With every turn of the cycle, one acetyl-CoA molecule enters, and a variety of molecules leave. These leaving molecules include ,
,
, and ATP. The acetyl-CoA that enters the cycle is derived from other cellular pathways, such as beta-oxidation and glycolysis. In this way, the citric acid cycle serves as a conduit by which metabolites from other pathways can be broken down to ultimately provide energy for the cell.
Since the citric acid cycle occurs in mitochondria, only eukaryotes are capable of performing this process. Also, the citric acid cycle is not responsible for the production of urea from ammonia. Rather, it is the urea cycle that performs this role. It is worth noting, however, that the citric acid cycle and the urea cycle are energetically linked by the aspartate-argininosuccinate shunt. This is because these two cycle share a few common intermediates, and the citric acid cycle can help to offset the demanding energy requirements of the urea cycle.
Example Question #1 : Glycogenolysis
Why is glycogen phosphorylase alone not sufficient in in degrading glycogen?
Glycogen phosphorylase can only cleave roughly ten bonds before it runs out of energy
Glycogen phosphorylase can only cleave one glycosidic bond at which point another glycogen phosphorylase enzyme must come cleave the next one
Glycogen phosphorylase can only cleave alpha-1,6-glycosidic bonds, and so when it reaches a branch point it stops
Glycogen phosphorylase can not cleave the alpha-1,6-glycosidic bonds at glycogen branch points
None of these
Glycogen phosphorylase can not cleave the alpha-1,6-glycosidic bonds at glycogen branch points
When glycogen phosphorylase reaches a branching point in glycogen, the bonds switch from being alpha-1,4-glycosidic bonds to alpha-1,6-glycosidic bonds. It is unable to cleave these bonds, and so other enzymes (a transferase and a glucosidase) must come into play.
Example Question #1 : Glycogenolysis Enzymes
Which enzymes are required for glycogen breakdown?
hexokinase, glycogen synthase, phosphoglucose isomerase
glycogen synthase, glycogen branching enzyme, UDP-glucose pyrophosphorylase
glycogen phosphorylase, glycogen branching enzyme, phosphoglutomutase
glycogen phosphorylase, glycogen debranching enzyme, phosphoglutomutase
glycogen synthase, glycogen debranching enzyme, UDP-glucose pyrophosphorylase
glycogen phosphorylase, glycogen debranching enzyme, phosphoglutomutase
Glycogen is first debranched and broken down from its non-reducing end by glycogen phosphorylase to give the product G1P, which is then converted into G6P by phosphoglutomutase. Glycogen synthase, glycogen branching enzyme, and UDP-glucose pyrophosphorylase are required for glycogen synthesis.
Example Question #1 : Alternative Pathways
What are some characteristics of glycogen phosphorylase?
I. It is the rate-limiting enzyme of glycogenolysis
II. It breaks alpha 1,4 glycosidic bonds
III. It is activated by epinephrine
IV. It breaks alpha 1,6 glycosidic bonds
II, III, and IV
I, II, and III
I and II
II and III
I and IV
I, II, and III
Glycogen phosphorylase, the rate-limiting enzyme of glycogenolysis does not breaks alpha 1,6 glycosidic bonds. It releases glucose from glycogen by hydrolyzing alpha 1,4 glycosidic bonds until it reaches a branch point in the glycogen molecule. At this time, another enzyme, a debranching alpha 1,6 glycosidase hydrolyzes the alpha 1,6 glycosidic bonds. Glycogen phosphorylase is under regulation by many hormones, including insulin and glucagon, as well as epinephrine.
Example Question #181 : Carbohydrate Metabolism
Which of the following enzymes is not required to breakdown glycogen into glucose-6-phosphate molecules for further metabolism?
All of these enzymes are necessary in the breakdown of glycogen into glucose-6-phosphate molecules.
Phosphoglucomutase
Glycogen phosphorylase
Glucosyltransferase
Alpha-1,6-glucosidase
All of these enzymes are necessary in the breakdown of glycogen into glucose-6-phosphate molecules.
In order to break down glycogen into individual glucose-6-phosphate units, all of the above enzymes are required. Each plays a specific role in one of the following activities: degradation of glycogen initially, remodeling of the glycogen so that it can be acted upon by the enzymes, and conversion of glucose-1-phosphate to glucose 6-phosphate.
Example Question #2 : Glycogenolysis
Which one of the following statements is incorrect?
Breakdown of glycogen in muscle produces mostly glucose, which is released into the blood.
Glycogen provides a reservoir of glucose molecules that can be used to replenish the blood with glucose when food is not available.
Glycogen provides a way to store energy in tissues that consume large amounts of energy when an organism is active.
Both the synthesis and the breakdown of glycogen are regulated.
Breakdown of glycogen in muscle produces mostly glucose, which is released into the blood.
Glycogen is mostly stored in the liver and skeletal muscle. When it is broken down in the liver, the last enzyme, a phosphatase, removes the last phosphate group to release plain glucose into the bloodstream. In the muscle, there is no need to release the glucose, so glycogen is only broken down as far as glucose-6-phosphate. Skeletal muscle cells lack the last phosphatase required to remove the phosphate from carbon 6. This isn't an obstacle, however, because the glucose-6-phosphate is already on to the second stage of glycolysis.
Example Question #2 : Alternative Pathways
Which one of the following statements is correct?
5’ AMP binds to muscle glycogen phosphorylase b and inhibits it by an allosteric mechanism.
Glucose stabilizes the R-state of liver glycogen phosphorylase a.
Glucagon stimulates conversion of muscle glycogen phosphorylase b to muscle glycogen phosphorylase a.
Insulin causes a liver cell to convert its glycogen phosphorylase a to glycogen phosphorylase b.
Insulin causes a liver cell to convert its glycogen phosphorylase a to glycogen phosphorylase b.
Insulin is released in response to high blood glucose. It causes a signaling cascade that, in addition to other things, stops glycogenolysis. This is done by converting glycogen phosphorylase from it's active "a" form to its inactive "b" configuration. The "R" state is the active state, so the presence of glucose would not trigger the breakdown of glycogen. 5' AMP would not inhibit an inactive form of an enzyme. High AMP would mean a demand for ATP, so it would convert the enzyme to its "a" form.
Example Question #1 : Alternative Pathways
Which of the following compounds is regenerated in the citric acid cycle?
Succinate
Oxaloacetate
Citrate
Pyruvate
Oxaloacetate
Oxaloacetate is the four-carbon molecule that is regenerated by the enzyme malate dehydrogenase in order to continue the cycle to form citrate with acetyl-CoA in the first step of the Krebs cycle. The other answer choices are intermediates of the citric acid cycle, but only oxaloacetate is regenerated, making it a true cycle.
Certified Tutor
Certified Tutor
All Biochemistry Resources
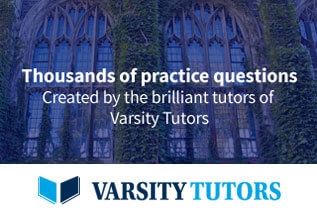