All ACT Science Resources
Example Questions
Example Question #181 : Physics
Sometimes scientists need to scale down experiments because collecting full-scale data may not be practical or possible. Most of the time, the data collected during a small scale-experiment is an accurate indicator of full-scale results. For example, research of craters on the Earth’s surface has indicated that the Earth has experienced a history of massive meteor collisions. It has been hypothesized that, amongst other things, these impacting meteorites caused the Earth’s oceans to vaporize. The detrimental effects associated with meteor collisions would have made the planet to be uninhabitable to humans. Due to these adverse effects, an experiment that could produce meteor-sized craters would not be possible in a full-scale setting.
Researchers have developed small-scale experiments that are exemplary of Newton’s three laws of motion. Newton’s first law states that an object in motion will stay in motion unless it is acted on by another force. This phenomenon is known as inertia. The second law comes in the form of an equation that calculates how much force is exerted on two colliding objects with respect to mass and acceleration. In other words force is equal to the mass of an object multiplied by its acceleration. The third law states that for every action there is an equal and opposite reaction. In other words, forces always occur in pairs. A counter force that is equal in magnitude and opposite in direction compliments each force in a particular direction. For example, if you push downwards on a table then the table, in theory, pushes upwards with the same force.
In a particular small-scale exercise, students were asked to design an experiment in which two “meteorites” would be dropped into a medium of sand and the subsequent crater diameters would be measured. Afterwards, the sand would be leveled and the experiment would be repeated. The students were asked to identify all of the factors that could affect the formation of the craters. This was done in order to ensure that each experiment was testing one independent and one dependent variable at a time. Otherwise, it would be unclear which variable is causing the observed effect. Possible variables that could alter the outcome of the experiment included but were not limited to the following: height of the fall, speed of the “meteorite,” angle of impact, and the mass of meteorite.
Consider an experiment that seeks to identify the mass of the meteorite analog. All other variables would have to remain constant in order to ensure accurate results. In order to do this, the height of the fall was set to one meter. The object was placed on top of a vertical meter stick. In order to keep the acceleration and height of the drop constant, it was rolled off of the stick instead of being pushed or simply dropped. Last, a protractor was used to keep the meter stick at a ninety-degree angle. In the procedure, a marble and a golf ball were used as “meteorites” of different masses.
The results of the experiment were recorded in the table and figure provided.
Crater Diameter (cm) | ||
Trial Number | ||
Marble (mass = 4g) | Golf ball (mass = 46g) | |
1 | 4.5 | 7.75 |
2 | 4.5 | 6 |
3 | 4 | 5.5 |
4 | 3 | 6 |
5 | 3 | 6.5 |
6 | 3.5 | 7 |
7 | 3 | 5 |
8 | 3 | 5 |
9 | 3 | 7 |
10 | 3.5 | 6 |
Average | Average | |
3.5 cm | 6.175 cm |
Many craters on Earth have already been found; however, scientists believe there are many more that will never be discovered. Which of the following represents the most significant factor supporting this theory?
Scientists have observed numerous amounts of craters on the surface of the moon
Burning fossil fuels
None of these
Erosion
Erosion
The massive number of craters found on the Earth supports the theory that states that the Earth was bombarded by a similar number of meteors as the moon; however, this theory does not explain why investigators will never discover those craters. Erosion is the most plausible answer. This is because erosion—in the form of human activities, hurricanes, vegetation growth, tsunamis, etc.—cause damage to craters that wears them down and makes some of them practically invisible.
Example Question #1302 : Act Science
Sometimes scientists need to scale down experiments because collecting full-scale data may not be practical or possible. Most of the time, the data collected during a small scale-experiment is an accurate indicator of full-scale results. For example, research of craters on the Earth’s surface has indicated that the Earth has experienced a history of massive meteor collisions. It has been hypothesized that, amongst other things, these impacting meteorites caused the Earth’s oceans to vaporize. The detrimental effects associated with meteor collisions would have made the planet to be uninhabitable to humans. Due to these adverse effects, an experiment that could produce meteor-sized craters would not be possible in a full-scale setting.
Researchers have developed small-scale experiments that are exemplary of Newton’s three laws of motion. Newton’s first law states that an object in motion will stay in motion unless it is acted on by another force. This phenomenon is known as inertia. The second law comes in the form of an equation that calculates how much force is exerted on two colliding objects with respect to mass and acceleration. In other words force is equal to the mass of an object multiplied by its acceleration. The third law states that for every action there is an equal and opposite reaction. In other words, forces always occur in pairs. A counter force that is equal in magnitude and opposite in direction compliments each force in a particular direction. For example, if you push downwards on a table then the table, in theory, pushes upwards with the same force.
In a particular small-scale exercise, students were asked to design an experiment in which two “meteorites” would be dropped into a medium of sand and the subsequent crater diameters would be measured. Afterwards, the sand would be leveled and the experiment would be repeated. The students were asked to identify all of the factors that could affect the formation of the craters. This was done in order to ensure that each experiment was testing one independent and one dependent variable at a time. Otherwise, it would be unclear which variable is causing the observed effect. Possible variables that could alter the outcome of the experiment included but were not limited to the following: height of the fall, speed of the “meteorite,” angle of impact, and the mass of meteorite.
Consider an experiment that seeks to identify the mass of the meteorite analog. All other variables would have to remain constant in order to ensure accurate results. In order to do this, the height of the fall was set to one meter. The object was placed on top of a vertical meter stick. In order to keep the acceleration and height of the drop constant, it was rolled off of the stick instead of being pushed or simply dropped. Last, a protractor was used to keep the meter stick at a ninety-degree angle. In the procedure, a marble and a golf ball were used as “meteorites” of different masses.
The results of the experiment were recorded in the table and figure provided.
Crater Diameter (cm) | ||
Trial Number | ||
Marble (mass = 4g) | Golf ball (mass = 46g) | |
1 | 4.5 | 7.75 |
2 | 4.5 | 6 |
3 | 4 | 5.5 |
4 | 3 | 6 |
5 | 3 | 6.5 |
6 | 3.5 | 7 |
7 | 3 | 5 |
8 | 3 | 5 |
9 | 3 | 7 |
10 | 3.5 | 6 |
Average | Average | |
3.5 cm | 6.175 cm |
This experiment was done using objects of differing masses. Instead, imagine that the experiment was done using objects of different volumes (i.e. the mass of the objects stayed the same but their size differed). What can we theorize about the craters?
The increase in volume will cause an increase in energy upon impact.
Because the mass does not change, the object will hit the ground with the same energy in every trial. This means that the craters will increase in diameter but the depth will decrease due to the energy spreading over a larger surface area.
Because the mass does not change, the object will hit the ground with the same energy in every trial. This means that the craters will decrease in diameter but the depth will increase due to the energy spreading over a larger surface area.
The increase in volume will cause a decrease in the energy expelled and the craters diameter will be smaller.
Because the mass does not change, the object will hit the ground with the same energy in every trial. This means that the craters will increase in diameter but the depth will decrease due to the energy spreading over a larger surface area.
The equation for kinetic energy does not account for surface area or volume because they are not factors that affect it. All objects free-fall at the same rate assuming there is no air resistance; therefore, if the mass remains the same, then the amount of energy will be kept constant. This also indicates that the same amount of energy is transferring to a larger area, which means that the objects will not travel as far into the medium.
Example Question #41 : How To Find Conflicting Viewpoints In Physics
Scientist 1: Scientist 1 believes that light displays particle behavior. This means that rays of light have their own associated momentum. Furthermore, Scientist 1 does not believe that light will exhibit wave behavior.
Scientist 2: Scientist 2 disagrees with Scientist 1 and believes that light can exhibit wave behavior, but does not display particle behavior. In other words, this scientist believes that light does not have any momentum.
Experiment: To settle their disagreement, the scientists setup the following experiment. The scientist take dark metallic material. This material is attached to pole and the metallic material can spin if it is subjected to a force; similar to a watermill or wind turbine. This setup is then placed outside and exposed to sunlight.
If the metallic surface rotates when exposed to light, whose viewpoint is strengthened and why?
Scientist 1, because the light particles do not display momentum
Cannot be determined from the given information
Scientist 1, because the light particles display momentum
Scientist 2, because the light particles display momentum
Scientist 2, because the light particles do not display momentum
Scientist 1, because the light particles display momentum
If the metallic surface is rotating this means that somewhat is striking the surface of the metallic material. Since the only thing that is hitting the metallic surface is sunlight, it indicates that the light particles have momentum. This supports Scientist 1's viewpoint.
Example Question #182 : Physics
Scientist 1: Scientist 1 believes that light displays particle behavior. This means that rays of light have their own associated momentum. Furthermore, Scientist 1 does not believe that light will exhibit wave behavior.
Scientist 2: Scientist 2 disagrees with Scientist 1 and believes that light can exhibit wave behavior, but does not display particle behavior. In other words, this scientist believes that light does not have any momentum.
Experiment: To settle their disagreement, the scientists setup the following experiment. The scientist take dark metallic material. This material is attached to pole and the metallic material can spin if it is subjected to a force; similar to a watermill or wind turbine. This setup is then placed outside and exposed to sunlight.
If during the experiment the metallic material does not move, whose viewpoint is strengthened and why?
Scientist 2, because the light particles do not display momentum
Scientist 2, because the light particles display momentum
Scientist 1, because the light particles display momentum
Cannot be determined from the given information
Scientist 1, because the light do not display momentum
Scientist 2, because the light particles do not display momentum
If the metallic surface does not move that implies that there is no force or any particles with momentum striking the surface. This means that the light particles are displaying wave behavior without momentum to strike the surface.
Example Question #183 : Physics
As part of an engineering competition, a group of students are asked to design a flying robot that simulates the way real birds fly. Below, three of the students give their explanations for how bird flight occurs.
Student 1:
Birds are able to fly due to the shape of their wings. Bird wings are convex on their upper sides, while their lower sides are usually concave. This type of shape is called an airfoil. When a wing travels through the air, air passing over the top of the wing must travel a greater distance than air passing under the wing. The stream of air passing over the wing and the stream of air passing under the wing meet together at the tail end of the wing. In order for both streams of air to meet at the same point behind the wing, the air above the wing, which travels a greater distance, must travel faster than the air below the wing.
When a volume of air travels more quickly over a distance, its molecules are spread out over a greater distance. As a result, the air traveling over the top of a wing has a lower pressure than the air traveling under the wing. Because the wing has a region of low pressure above it and a region of relative high pressure below it, it experiences a net upward force. When this upward force is greater than or equal to the bird's weight, or the force exerted on a bird by gravity, the bird is able to fly.
The magnitude of the upward force depends on the speed at which air flows across the wing and on the corresponding difference in pressure over and under the wing. When birds flap their wings, they increase the speed of air flowing across their wings, thus producing a greater upward force.
Student 2:
There are two components to bird flight: lift and thrust. "Lift" refers to the upward force that allows a bird to stay aloft in the air despite its weight, while "thrust" refers to the horizontal force that allows a bird to move forward through the air. Birds are able to fly because they do not hold their wings perfectly horizontally. Instead, their wings are angled slightly upward. The angle at which a wing is inclined upward, with respect to the horizontal, is called its "angle of attack."
Air is not an ideal gas; instead, it has viscosity. This means that the air flowing close to a solid object tends to follow the curves of that object. When air encounters a bird's wing, it follows the incline of the wing. Because of the wing's angle of attack, the air is directed downward and back. The air continues to move downward, even after it has left the wing. This movement of the air creates an opposing force that pushes the bird upward and forward.
Thus, the angle of attack of a bird's wings accounts for both the lift and thrust components of a bird's flight.
Student 3:
Birds are able to fly because the way in which they move their wings allows them to create a net movement of air downward and backward. The flapping of a bird's wings can be understood as being composed of two parts: a downstroke, during which the bird moves its wings down, and an upstroke, during which the bird moves its wings up. During a downstroke, a bird displaces a quantity of air downward and behind it. During an upstroke, however, the bird's wings are angled upward in a way that displaces less air, and its wing feathers rotate to allow air to pass through them. Thus, on the upstroke, the bird much exerts less force on the air than it does on the downstroke.
The explanation given by Student 3 differs from the explanation given by Student 2 in that __________.
Student 3 explains flight in terms of a wing's downstroke and upstroke, whereas Student 2 explains flight in terms of how the shape of a wing directs air
Student 3 does not explain how birds produce thrust, whereas Student 2 does
Student 3's explanation can be used to understand both flapping and non-flapping flight, while Student 2's explanation applies only to flight where birds do not flap their wings
Student 3 states that the force generated by a wing is determined by the speed at which it moves, while Student 2 states that the force generated by a wing is determined by how air flows along the surface of the wing
Student 3 explains flight in terms of a wing's downstroke and upstroke, whereas Student 2 explains flight in terms of how the shape of a wing directs air
Both Student 2 and Student 3 explain flight in terms of equal and opposite forces that are generated when wings move air downward and backward; however, Student 2 states that air moves downward and backward because it follows the shape and angle of a wing, while Student 3 states that air moves downward and backward because it is pushed down by a wing during the wing's downstroke.
Example Question #181 : Physics
As part of an engineering competition, a group of students are asked to design a flying robot that simulates the way real birds fly. Below, three of the students give their explanations for how bird flight occurs.
Student 1:
Birds are able to fly due to the shape of their wings. Bird wings are convex on their upper sides, while their lower sides are usually concave. This type of shape is called an airfoil. When a wing travels through the air, air passing over the top of the wing must travel a greater distance than air passing under the wing. The stream of air passing over the wing and the stream of air passing under the wing meet together at the tail end of the wing. In order for both streams of air to meet at the same point behind the wing, the air above the wing, which travels a greater distance, must travel faster than the air below the wing.
When a volume of air travels more quickly over a distance, its molecules are spread out over a greater distance. As a result, the air traveling over the top of a wing has a lower pressure than the air traveling under the wing. Because the wing has a region of low pressure above it and a region of relative high pressure below it, it experiences a net upward force. When this upward force is greater than or equal to the bird's weight, or the force exerted on a bird by gravity, the bird is able to fly.
The magnitude of the upward force depends on the speed at which air flows across the wing and on the corresponding difference in pressure over and under the wing. When birds flap their wings, they increase the speed of air flowing across their wings, thus producing a greater upward force.
Student 2:
There are two components to bird flight: lift and thrust. "Lift" refers to the upward force that allows a bird to stay aloft in the air despite its weight, while "thrust" refers to the horizontal force that allows a bird to move forward through the air. Birds are able to fly because they do not hold their wings perfectly horizontally. Instead, their wings are angled slightly upward. The angle at which a wing is inclined upward, with respect to the horizontal, is called its "angle of attack."
Air is not an ideal gas; instead, it has viscosity. This means that the air flowing close to a solid object tends to follow the curves of that object. When air encounters a bird's wing, it follows the incline of the wing. Because of the wing's angle of attack, the air is directed downward and back. The air continues to move downward, even after it has left the wing. This movement of the air creates an opposing force that pushes the bird upward and forward.
Thus, the angle of attack of a bird's wings accounts for both the lift and thrust components of a bird's flight.
Student 3:
Birds are able to fly because the way in which they move their wings allows them to create a net movement of air downward and backward. The flapping of a bird's wings can be understood as being composed of two parts: a downstroke, during which the bird moves its wings down, and an upstroke, during which the bird moves its wings up. During a downstroke, a bird displaces a quantity of air downward and behind it. During an upstroke, however, the bird's wings are angled upward in a way that displaces less air, and its wing feathers rotate to allow air to pass through them. Thus, on the upstroke, the bird much exerts less force on the air than it does on the downstroke.
Suppose that a robot is designed with a pair of wings that, on their downstroke, displace air downward and backward with a force of 10 N, and that, on their upstroke, displace air upward and forward with a force of 5 N.
Given that Student 3's explanation is correct, is it possible for such a robot to fly?
Yes, it is possible, because the robot's wings exert a net force upward and forward on the air.
No, it is not possible, because the robot's wings exert a net force downward and backward on the air.
Yes, it is possible, because the robot's wings exert a net force downward and backward on the air.
No, it is not possible, because the robot's wings exert a net force upward and forward on the air.
Yes, it is possible, because the robot's wings exert a net force downward and backward on the air.
Student 3 states that birds are able to fly because their wings exert relatively more downward and backward force on air than they do forward and upward. According to Student 3, when a bird's wings push air backward and downward, the bird experiences an opposing force that carries it upward and forward.
In the situation described in this problem, the robot does exert a net downward and backward force on the air. So, it is possible for the robot to fly, because it will experience a force that carries it upward and forward.
Example Question #181 : Physics
As part of an engineering competition, a group of students are asked to design a flying robot that simulates the way real birds fly. Below, three of the students give their explanations for how bird flight occurs.
Student 1:
Birds are able to fly due to the shape of their wings. Bird wings are convex on their upper sides, while their lower sides are usually concave. This type of shape is called an airfoil. When a wing travels through the air, air passing over the top of the wing must travel a greater distance than air passing under the wing. The stream of air passing over the wing and the stream of air passing under the wing meet together at the tail end of the wing. In order for both streams of air to meet at the same point behind the wing, the air above the wing, which travels a greater distance, must travel faster than the air below the wing.
When a volume of air travels more quickly over a distance, its molecules are spread out over a greater distance. As a result, the air traveling over the top of a wing has a lower pressure than the air traveling under the wing. Because the wing has a region of low pressure above it and a region of relative high pressure below it, it experiences a net upward force. When this upward force is greater than or equal to the bird's weight, or the force exerted on a bird by gravity, the bird is able to fly.
The magnitude of the upward force depends on the speed at which air flows across the wing and on the corresponding difference in pressure over and under the wing. When birds flap their wings, they increase the speed of air flowing across their wings, thus producing a greater upward force.
Student 2:
There are two components to bird flight: lift and thrust. "Lift" refers to the upward force that allows a bird to stay aloft in the air despite its weight, while "thrust" refers to the horizontal force that allows a bird to move forward through the air. Birds are able to fly because they do not hold their wings perfectly horizontally. Instead, their wings are angled slightly upward. The angle at which a wing is inclined upward, with respect to the horizontal, is called its "angle of attack."
Air is not an ideal gas; instead, it has viscosity. This means that the air flowing close to a solid object tends to follow the curves of that object. When air encounters a bird's wing, it follows the incline of the wing. Because of the wing's angle of attack, the air is directed downward and back. The air continues to move downward, even after it has left the wing. This movement of the air creates an opposing force that pushes the bird upward and forward.
Thus, the angle of attack of a bird's wings accounts for both the lift and thrust components of a bird's flight.
Student 3:
Birds are able to fly because the way in which they move their wings allows them to create a net movement of air downward and backward. The flapping of a bird's wings can be understood as being composed of two parts: a downstroke, during which the bird moves its wings down, and an upstroke, during which the bird moves its wings up. During a downstroke, a bird displaces a quantity of air downward and behind it. During an upstroke, however, the bird's wings are angled upward in a way that displaces less air, and its wing feathers rotate to allow air to pass through them. Thus, on the upstroke, the bird much exerts less force on the air than it does on the downstroke.
The amount of force exerted on an object can be calculated by multiplying the mass of the object by the acceleration it experiences. Given that Student 3's explanation is correct, which of the following situations would allow a bird's body to experience the greatest upward force?
The bird's wings displace of air downward at an acceleration of
.
The bird's wings displace of air downward at an acceleration of
.
The bird's wings displace of air upward at an acceleration of
.
The bird's wings displace of air upward at an acceleration of
.
The bird's wings displace of air downward at an acceleration of
.
According to Student 3, when a bird's wings push air backward and downward, the bird experiences an opposing force that carries it upward and forward. So, when a bird's wings exert a greater downward force on air, the bird will experience a greater force pulling it upward.
Since force is calculated by multiplying the mass of the air displaced by the acceleration that the air experiences, the greatest force is exerted when of air is displaced at an acceleration of
.
Example Question #1306 : Act Science
As part of an engineering competition, a group of students are asked to design a flying robot that simulates the way real birds fly. Below, three of the students give their explanations for how bird flight occurs.
Student 1:
Birds are able to fly due to the shape of their wings. Bird wings are convex on their upper sides, while their lower sides are usually concave. This type of shape is called an airfoil. When a wing travels through the air, air passing over the top of the wing must travel a greater distance than air passing under the wing. The stream of air passing over the wing and the stream of air passing under the wing meet together at the tail end of the wing. In order for both streams of air to meet at the same point behind the wing, the air above the wing, which travels a greater distance, must travel faster than the air below the wing.
When a volume of air travels more quickly over a distance, its molecules are spread out over a greater distance. As a result, the air traveling over the top of a wing has a lower pressure than the air traveling under the wing. Because the wing has a region of low pressure above it and a region of relative high pressure below it, it experiences a net upward force. When this upward force is greater than or equal to the bird's weight, or the force exerted on a bird by gravity, the bird is able to fly.
The magnitude of the upward force depends on the speed at which air flows across the wing and on the corresponding difference in pressure over and under the wing. When birds flap their wings, they increase the speed of air flowing across their wings, thus producing a greater upward force.
Student 2:
There are two components to bird flight: lift and thrust. "Lift" refers to the upward force that allows a bird to stay aloft in the air despite its weight, while "thrust" refers to the horizontal force that allows a bird to move forward through the air. Birds are able to fly because they do not hold their wings perfectly horizontally. Instead, their wings are angled slightly upward. The angle at which a wing is inclined upward, with respect to the horizontal, is called its "angle of attack."
Air is not an ideal gas; instead, it has viscosity. This means that the air flowing close to a solid object tends to follow the curves of that object. When air encounters a bird's wing, it follows the incline of the wing. Because of the wing's angle of attack, the air is directed downward and back. The air continues to move downward, even after it has left the wing. This movement of the air creates an opposing force that pushes the bird upward and forward.
Thus, the angle of attack of a bird's wings accounts for both the lift and thrust components of a bird's flight.
Student 3:
Birds are able to fly because the way in which they move their wings allows them to create a net movement of air downward and backward. The flapping of a bird's wings can be understood as being composed of two parts: a downstroke, during which the bird moves its wings down, and an upstroke, during which the bird moves its wings up. During a downstroke, a bird displaces a quantity of air downward and behind it. During an upstroke, however, the bird's wings are angled upward in a way that displaces less air, and its wing feathers rotate to allow air to pass through them. Thus, on the upstroke, the bird much exerts less force on the air than it does on the downstroke.
Because the bird exerts a net downward and backward force on the air, it experiences an equal and opposite force which carries it upward and forward.
Student 2's account of the the way that a flow of air propels a bird forward is most similar to which of the following situations?
A glider gains speed as it circles around a column of rising air.
A ping-pong ball is lifted up in the stream of hot air from an upturned blow-dryer.
A kite is blown north by a breeze coming from the south.
An airplane's propellers push the airplane forward by blowing air behind the airplane.
An airplane's propellers push the airplane forward by blowing air behind the airplane.
In the explanation, Student 2 states that a bird's wing propels a bird forward by directing air downward and backward. This is most similar to the way that an airplane moves forward when its propellers blow air backward. In both cases, a flying object propels itself forward by moving a medium in the opposite direction.
Example Question #51 : How To Find Conflicting Viewpoints In Physics
As part of an engineering competition, a group of students are asked to design a flying robot that simulates the way real birds fly. Below, three of the students give their explanations for how bird flight occurs.
Student 1:
Birds are able to fly due to the shape of their wings. Bird wings are convex on their upper sides, while their lower sides are usually concave. This type of shape is called an airfoil. When a wing travels through the air, air passing over the top of the wing must travel a greater distance than air passing under the wing. The stream of air passing over the wing and the stream of air passing under the wing meet together at the tail end of the wing. In order for both streams of air to meet at the same point behind the wing, the air above the wing, which travels a greater distance, must travel faster than the air below the wing.
When a volume of air travels more quickly over a distance, its molecules are spread out over a greater distance. As a result, the air traveling over the top of a wing has a lower pressure than the air traveling under the wing. Because the wing has a region of low pressure above it and a region of relative high pressure below it, it experiences a net upward force. When this upward force is greater than or equal to the bird's weight, or the force exerted on a bird by gravity, the bird is able to fly.
The magnitude of the upward force depends on the speed at which air flows across the wing and on the corresponding difference in pressure over and under the wing. When birds flap their wings, they increase the speed of air flowing across their wings, thus producing a greater upward force.
Student 2:
There are two components to bird flight: lift and thrust. "Lift" refers to the upward force that allows a bird to stay aloft in the air despite its weight, while "thrust" refers to the horizontal force that allows a bird to move forward through the air. Birds are able to fly because they do not hold their wings perfectly horizontally. Instead, their wings are angled slightly upward. The angle at which a wing is inclined upward, with respect to the horizontal, is called its "angle of attack."
Air is not an ideal gas; instead, it has viscosity. This means that the air flowing close to a solid object tends to follow the curves of that object. When air encounters a bird's wing, it follows the incline of the wing. Because of the wing's angle of attack, the air is directed downward and back. The air continues to move downward, even after it has left the wing. This movement of the air creates an opposing force that pushes the bird upward and forward.
Thus, the angle of attack of a bird's wings accounts for both the lift and thrust components of a bird's flight.
Student 3:
Birds are able to fly because the way in which they move their wings allows them to create a net movement of air downward and backward. The flapping of a bird's wings can be understood as being composed of two parts: a downstroke, during which the bird moves its wings down, and an upstroke, during which the bird moves its wings up. During a downstroke, a bird displaces a quantity of air downward and behind it. During an upstroke, however, the bird's wings are angled upward in a way that displaces less air, and its wing feathers rotate to allow air to pass through them. Thus, on the upstroke, the bird much exerts less force on the air than it does on the downstroke.
Which of the following questions is not answered in the explanation of flight given by Student 1?
How does the shape of a bird's wing allow the bird to fly?
What determines the strength of the upward force that a bird experiences?
What happens to the force a bird experiences when the bird flaps its wings?
How can a bird remain in the air without flapping its wings?
How does a bird move forward through the air?
How does a bird move forward through the air?
Student 1 explains birds' ability to fly in terms of the airfoil shape of their wings. This explanation states that by creating a region of relative low pressure above the wing and relative high pressure below the wing, birds' wings produce an upward force which acts on the bird; however, this explanation does not discuss any forward force acting on a bird. This explanation only gives an account of how birds stay aloft in the air, not of how they move forward.
Example Question #191 : Physics
As part of an engineering competition, a group of students are asked to design a flying robot that simulates the way real birds fly. Below, three of the students give their explanations for how bird flight occurs.
Student 1:
Birds are able to fly due to the shape of their wings. Bird wings are convex on their upper sides, while their lower sides are usually concave. This type of shape is called an airfoil. When a wing travels through the air, air passing over the top of the wing must travel a greater distance than air passing under the wing. The stream of air passing over the wing and the stream of air passing under the wing meet together at the tail end of the wing. In order for both streams of air to meet at the same point behind the wing, the air above the wing, which travels a greater distance, must travel faster than the air below the wing.
When a volume of air travels more quickly over a distance, its molecules are spread out over a greater distance. As a result, the air traveling over the top of a wing has a lower pressure than the air traveling under the wing. Because the wing has a region of low pressure above it and a region of relative high pressure below it, it experiences a net upward force. When this upward force is greater than or equal to the bird's weight, or the force exerted on a bird by gravity, the bird is able to fly.
The magnitude of the upward force depends on the speed at which air flows across the wing and on the corresponding difference in pressure over and under the wing. When birds flap their wings, they increase the speed of air flowing across their wings, thus producing a greater upward force.
Student 2:
There are two components to bird flight: lift and thrust. "Lift" refers to the upward force that allows a bird to stay aloft in the air despite its weight, while "thrust" refers to the horizontal force that allows a bird to move forward through the air. Birds are able to fly because they do not hold their wings perfectly horizontally. Instead, their wings are angled slightly upward. The angle at which a wing is inclined upward, with respect to the horizontal, is called its "angle of attack."
Air is not an ideal gas; instead, it has viscosity. This means that the air flowing close to a solid object tends to follow the curves of that object. When air encounters a bird's wing, it follows the incline of the wing. Because of the wing's angle of attack, the air is directed downward and back. The air continues to move downward, even after it has left the wing. This movement of the air creates an opposing force that pushes the bird upward and forward.
Thus, the angle of attack of a bird's wings accounts for both the lift and thrust components of a bird's flight.
Student 3:
Birds are able to fly because the way in which they move their wings allows them to create a net movement of air downward and backward. The flapping of a bird's wings can be understood as being composed of two parts: a downstroke, during which the bird moves its wings down, and an upstroke, during which the bird moves its wings up. During a downstroke, a bird displaces a quantity of air downward and behind it. During an upstroke, however, the bird's wings are angled upward in a way that displaces less air, and its wing feathers rotate to allow air to pass through them. Thus, on the upstroke, the bird much exerts less force on the air than it does on the downstroke.
Suppose that a robot is designed with stationary wings in the shape of airfoils; however, it is found that the robot is still not able to fly. Which of the following suggestions would Student 1 most likely not make about how to change the design of the robot?
The angle of attack of the robot's wings should be increased.
The robot's weight should be decreased.
The robot should be designed to flap its wings.
The shape of the wings should be improved to make them more efficient airfoils.
The angle of attack of the robot's wings should be increased.
In the explanation, Student 1 does not mention the degree of the angle of attack of wings as a factor in determining whether or not a bird is able to fly. So, Student 1 would likely not suggest that the robot's wings' angle of attack should be increased; however, Student 1 does mention weight, the air-pressure difference generated by the shape of wings, and flapping as factors that affect flight.
Certified Tutor
All ACT Science Resources
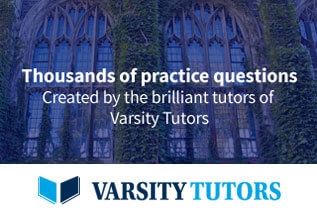